Thermally conductive potting compounds make way for higher power density electronics
Now more than ever, we are seeing a strong trend toward the electrification of vehicles in the transportation industry. In these electric vehicles, long ranges and high horsepower require high power density from their electrical components such as batteries, motors and generators, and the power electronics needed for operation. The trend is focused on smaller, less expensive components that will save space and reduce costs while boosting power efficiency.
A key challenge in developing these components is to manage the heat generated by smaller, high-power devices. Thermally conductive potting compounds are ideal for rapidly and effectively conducting heat away from power components to the heat sink. The potting compound fills the component enclosure, leaving no air gaps. As a result, heat is dissipated within the enclosure and rapidly conducted to the heat sink, which has enabled substantial size reductions of the finished component.
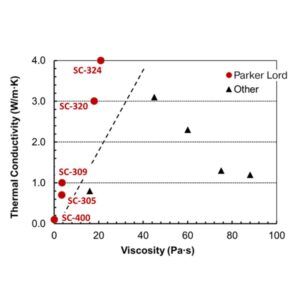
In a recent Parker Lord white paper, Thermally Conductive Potting Compounds Enable Higher Power Density Electronics, they share the results of a study conducted by a team from Parker Lord and Manzanita Micro who tested the heat rise of inductors potted in a liquid-cooled aluminum fixture.
In this study, they used five silicone potting materials—all currently used in numerous applications to protect sensitive electronic components from excess heat. These potting materials include Thermoset SC-400 Silicone Encapsulant and CoolTherm SC-305, SC-309, SC-320 and SC-324 Thermally Conductive Silicone Encapsulants. All the materials are electrically insulative with high dielectric strength and high-temperature stability.
A key differentiating property of these thermally conductive silicone materials is their low viscosity compared to competitive materials with similar thermal conductivity. The lower viscosity allows these materials to flow and fill voids more readily while providing excellent thermal conductivity. They are also easier to degas, meaning that parts can be potted that are essentially void-free if a vacuum potting process is used.
The inductors used in this study were made from Micrometals T400-61D high-temperature, magnetic-powder toroid cores wrapped with 62 turns of 10-gauge insulated copper wire and yielding about 500μH inductance at load. Inductors were potted in custom-made aluminum cooling plates fitted with liquid inlet and outlet ports for connection to an external circulating fluid bath. The materials were used to pot three inductors with each material, resulting in a total of 15 potted inductors. Before potting, each inductor was fitted with a thermocouple to measure its temperature.
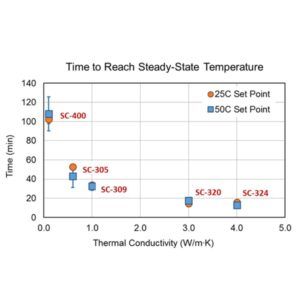
For the thermal tests, each inductor was connected to a Manzanita Micro PFC40X-188 charger. The aluminum cooling plate was connected to a temperature-controlled liquid bath; each inductor was tested at coolant temperature set points of 25°C and the typical automotive coolant temperature of 50°C. A second thermocouple was attached to the exterior of the cooling plate to monitor temperature changes during the test; however, the temperature rise of the cooling plate was less than 2°C, even in the most extreme cases.
During each test, temperatures of the inductor and cooling plate were recorded. The cooling plate and inductor temperatures were allowed to stabilize with no power. Power was then applied, and the temperature was monitored until the inductor temperature stabilized. Equilibrium was established at the point when the temperature reached a steady state, and the temperature rise was calculated as the final inductor temperature minus the initial temperature.
The data show that both the inductor temperature rise and the equilibration time are independent of the coolant temperature, as the data is within the test variation. And, the variation becomes much smaller as the thermal conductivity increases due to the more effective thermal connection to the aluminum cooling plate.
Comparing an inductor potted with an insulating material to the most conductive material, the heat rise is decreased by about 50°C, and the time required to reach a stable temperature is decreased from nearly two hours to 15 minutes. These significant improvements in heat management enable the development of significantly smaller power electronics.
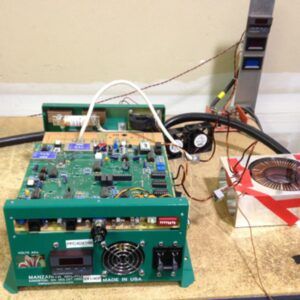
Dramatic reductions in temperature rise and equilibration time were observed for inductors potted with thermally conductive materials. The average temperature rise with Parker Lord’s Thermoset SC-400 encapsulant was about 55°C, and the average rise for the company’s most thermally conductive materials, CoolTherm SC-320 and SC-324 encapsulants, was less than 10°C. Even the moderately conductive silicones, CoolTherm SC-305 and SC-309 encapsulants provided significant improvements. With the non-conductive silicone, Thermoset SC-400 encapsulant, nearly two hours were required to reach a steady state, whereas a stable temperature was achieved in less than 20 minutes for CoolTherm SC-320 and SC-324 encapsulants. Under different test conditions generating a temperature rise much greater than 55°C, the improvement would likely be even more pronounced.
This rapid temperature recovery means that heat is dissipated quickly, and components spend less time at elevated temperatures, thus increasing the lifetime of the components.
Proper thermal management is essential for developing power electronics that are smaller and lighter and therefore, more power dense. As the study proves, using thermal management materials provides the unique combination of high thermal conductivity and low viscosity as well as substantial reductions in both maximum temperature rise and the time to reach a stable temperature.