Known as the birthplace of the British transport industry, it’s fitting that Coventry is proving to be a key location for it moving into a sustainable post-ICE future. With UK£3 million invested over the last two years, Coventry University and FEV’s Centre for Advanced Low-Carbon Systems (C-ALPS) is a state-of-art hydrogen research, development and testing facility designed to support UK companies in meeting future propulsion technology demands.
As our climate worsens, decarbonization becomes an increasingly pressing imperative for aircraft, automotive and off-highway machine manufacturers. Coventry University and engineering services provider FEV co-created the Centre for Advanced Low-Carbon Systems (C-ALPS) to develop the varied propulsion technologies this common endeavor demands. The research, testing and development facility works with leading engine and vehicle manufacturers, electrification start-ups and UK and European research consortia to develop new solutions for e-mobility and zero-emission drive systems.
“We have five propulsion test-cells, initially designed to test combustion engines and upgraded to support other low-carbon powertrains,” says C-ALPS Director, Dr Simon Shepherd. “Each has gasoline, diesel and hydrogen fuel supply and battery emulation capability. Dynamometers provide a mechanical load, or we can power an electric drive test bench. The cells have process water coolant and air extraction for exhaust fumes or leaked hydrogen.”
FEV primarily use these test-cells for contract powertrain testing, while specialized laboratories accommodate fundamental component and subsystem research. There are laboratories for high- and low-voltage electronics, hydrogen testing and cell prototyping.
“We do battery characterization and research into diagnostic techniques,” says Shepherd. “We want to understand the levers to improving battery performance, whether through faster charging or improved lifetime, energy-density or power-density.”
C-ALPS creates an unfenced continuum of academic and commercial research. Coventry University has a history of facilities like the Institute for Advanced Manufacturing and Engineering based on strong industrial partnerships. In 1978, FEV started life as an offshoot of Germany’s Aachen University, where it maintains close research collaboration.
“By 2013, we sought a similar university collaboration to serve our UK customers,” explains FEV UK Managing Director, Dr Jörn Behrenroth. “Coventry had ambition and consider themselves a disruptor. For German companies, building in the UK is hard, but it’s what the University does. We share facilities, co-ordinate investment and drive the topics we want to work on.”
FEVs long-term tenancy gave Coventry confidence to invest in propulsion system test capabilities at a scale beyond most universities. FEV co-funds PhD studies and underwrites the industrial relevance of academic research.
“Our international operations mean we understand industry needs and can provide industrial data,” says Behrenroth. “A PhD student developing a battery optimization control algorithm can spend months creating a model to test the algorithm. FEV already has those models, so they can focus on the actual solution.”
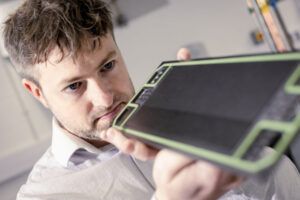
Scaling up
First conceived as an engine testbed, C-ALPS evolved to accommodate electrification projects. By 2020, it was clear that hydrogen, too, had a role in transport decarbonization and the partners invested £3m over two years in hydrogen testing capabilities.
“Coventry University funded conversion of one test cell to show it made sense,” Behrenroth recalled. “FEV followed up with a major upgrade of the other cells. It’s sometimes hard for commercial organizations to make an upfront investment case. Now, we can start with a small, tentative step to test the market.”
Already, C-ALPS has secured £4m in hydrogen testing contracts and committed to a long-term supply of hydrogen in industrial quantities from Element 2, benefitting both commercial and academic research. Completed in 2021, a 100m2 fuel cell laboratory enables cost-effective short-stack research.
“A short, 10kW fuel cell stack may be a subset of the 100kW stack needed for vehicle propulsion,” Shepherd explains. “It allows subscale testing of new materials or core performance in different conditions at reduced cost prior to scaling up.”
Dr Oliver Curnick leads Coventry University’s Hydrogen Energy Research Group, which studies both hydrogen propulsion and production. Curnick sees hydrogen conversion as primarily a challenge of scaling, which the partnership with FEV enables Coventry scientists to realistically address.
“University labs usually have subscale systems,” says Curnick. “Fuel-cells electrodes may be 25cm2. FEV’s more capable infrastructure facilitates testing at application scale, with several hundred cm2 electrodes, making our research relevant to scale-up challenges facing the industry. We’re developing in-situ sensors to measure oxygen concentrations inside fuel cells. Evaluation at proper scale shows us how to improve their performance in real systems.”
Coventry University works with industrial partners on FEV-led projects and through its own direct contracts. C-ALPS battery prototyping facilities enable academics to fabricate and test new cell technologies in automotive application formats. C-ALPS participates in government- or EU-funded collaborative projects such as CoacHyfied, to which FEV involvement maintains good post-Brexit access.
“We have PhD research studies fully or part-funded by industrial partners, with access to some facilities,” says Shepherd. “Those have a slower drumbeat but can kick-start ideas with low investment. We can have a student working on a tiny scooter motor and a full-scale commercial engine test in adjacent rooms.”
The C-ALPS approach is multi-sector and technology-agnostic. While hydrogen may prove apt for zero-emissions coaches and trucks, passenger cars seem set on a course of electrification. Here, work is focused on improving the practicality of battery-electric vehicles (BEVs) by enabling rapid charging without compromising battery lifetime.
“Battery prototyping helps customers look at new materials,” says Shepherd. “Developing advanced sensing techniques to monitor and understand the internal parameters of batteries could enable us to better model and manage them in use.”
Battery and hydrogen propulsion systems must aspire to the maturity of traditional engine technology optimized over a hundred years. Today, BEV owners are advised not to fully charge and effectively carry 20% spare capacity just to safeguard battery lifetime.
“Engine testbeds measure cylinder pressure, internal temperatures and model swirl-patterns,” says Behrenroth. “We want to do the same for other technologies. In-situ sensors may reveal how electrochemical processes impact cell longevity and enable smaller, lighter batteries to operate with less safety-margin. The University is developing a microprocessor to distribute internal sensor information to a battery management system.”
Novel in-situ sensors will illuminate processes internal to both batteries and fuel cells. By 2040, FEV projects that 86% of passenger cars will be battery-electric, 12% powered by fuels cells and 2% by hydrogen combustion. Curnick anticipates a niche market for hydrogen cars.
“It may be limited to luxury vehicles requiring expensive battery-packs,” he says. “People may pay a premium for the convenience in refueling a hydrogen-powered variant. The main barrier is absence of refueling infrastructure, but that is being rolled out in mainland Europe.”
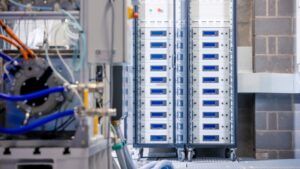
Hydrogen help
FEV predicts only 56% of off-highway machines will be fully decarbonized by 2040, with 38% employing batteries, 15% combusting hydrogen and only 3% using fuel cells. Batteries may be a good fit for mini excavators with urban or indoor applications, with large machines more apt for hybrid or hydrogen propulsion.
Heavy battery-packs might be accommodated on machines like excavators which are already counterweighted. But downtime costs money and a backhoe loader’s duty-cycle may not admit several hours’ break for charging. Recharging several machines could soon overload the grid and many work in remote locations with no grid connection.
“Digging a tunnel in Warwickshire for HS2, there will be no power,” says Behrenroth. “As an energy-vector, hydrogen could get you there. We have a hydrogen trailer feeding our test-cells, which we change every fortnight. A similar model could work for remote worksites.”
JCB recently showcased such a supply vehicle in conjunction with its hydrogen-powered demonstration backhoe loader and telehandler. Both use hydrogen combustion engines, which are a robust and market-ready solution.
“Airborne particulates on worksites may cause problems for fuel cells,” says Curnick. “Hydrogen is a new fuel, but the underlying combustion engine concept is the same. We know the degradation modes and have well-defined accelerated stress tests to determine durability. Hydraulic systems already driven by combustion engines can also be translated without modification.”
Just as construction machines work long shifts, so aircraft fulfil extended mission profiles. While batteries may power urban air-taxis, Shepherd perceives a consensus that hydrogen turbines or fuel cells must propel larger, regional aircraft. C-ALPS can test fuel cell powertrains to 500kW-scale and short-stack tests could deliver power-density increases to meet volumetric and weight constraints in aircraft design.
“Electric vertical takeoff aircraft may benefit from cross-fertilization with validated automotive powertrain optimization,” says Behrenroth. “We do commercial cell-testing for aerospace manufacturers. We can put a powertrain with fuel cell and e-machine in a test-cell, apply a load and run powertrain-in-the-loop simulations to de-risk development.”
Curnick doubts the sufficiency of hydrogen storage for long-haul flights on wide-body aircraft. Currently, he believes converted jet engines offers the most viable hydrogen propulsion system but expects fuel cell systems to exceed the power-density of turbines and become viable for smaller aircraft by the mid-2030s.
“Even light aircraft will probably need liquid hydrogen to achieve required power-densities,” says Curnick. “Therefore, we must develop methods and technologies for handling hydrogen at cryogenic temperatures – transfer lines, compressors, dryers, flow-meters – robust and reliable enough for commercial aerospace.”
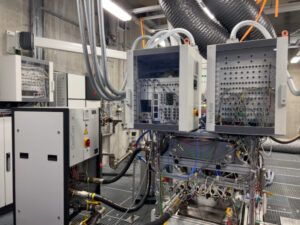
Future skills
C-ALPS research into hydrogen production explores novel technologies for improved efficiency of energy-intensive hydrogen compression. Some 95% of UK-produced hydrogen is derived from methane, a greenhouse gas 30 times more potent than CO2 and undesirable in the energy system. C-ALPS aspires to develop efficient means of electrolysis yielding more hydrogen per kilowatt-hour of electricity.
“The fundamental source of renewable energy underlies any mix of technologies, whether we use it to make hydrogen or charge batteries,” says Shepherd. “The roadmap to that doesn’t yet exist, so building the hydrogen economy is a chicken-or-egg problem. Nobody will buy hydrogen vehicles with nowhere to refuel them – so investors see no market for hydrogen production.”
Shortage of relevant expertise presents a major impediment to the development of battery and hydrogen propulsion systems, which is why Coventry University is fast-tracking relevant skills delivery to address this shortfall.
“Rapid transition away from combustion engines is creating opportunities,” says Shepherd. “There are new players and OEMs trying to shift their technology. But everyone is struggling to find people with skills to support the ambition. We’re transitioning our degree programs and offering our industrial partners short courses to retrain engineers in these new technologies.”
Transfixed by the magic of electrolysis as an undergraduate, Curnick has seen successive cycles of hype and disillusionment around hydrogen. This time, he believes it will stick, but still sees commercial and technical risk to developing propulsion systems or electrolytic production capacity.
“Development needs to be supported by clear government policy indicators to provide long-term certainty,” he says. “The Potsdam Institute recently compared historical scale-up rates of different technologies. It found a 6,000-fold increase in electrolytic capacity would be needed to reach net zero by 2050. The only technology in history to scale faster than that was Covid-19 vaccines.”